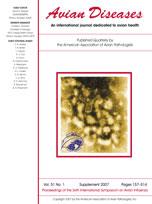
No abstract available
No abstract available
No abstract available
Virologic Findings in Selected Free-Range Mule Duck Farms at High Risk for Avian Influenza Infection